CSC/ECE 506 Spring 2015/7a ap: Difference between revisions
Line 187: | Line 187: | ||
Following are the detailed explanation of consistency models<ref>http://en.wikipedia.org/wiki/Consistency_model</ref>: | Following are the detailed explanation of consistency models<ref>http://en.wikipedia.org/wiki/Consistency_model</ref>: | ||
====Atomic Consistency==== | ====Atomic or Strict Consistency==== | ||
<ref>http://en.wikipedia.org/wiki/Linearizability</ref> | <ref>http://en.wikipedia.org/wiki/Linearizability</ref> | ||
This model is the strictest of all consistency models. The model works on the concept of atomicity. In this model, all the memory accesses are performed atomically that is one memory access from any one processor can be executed at any given time and secondly the whole sequence of memory accesses are executed entirely. They can not be interrupted by any concurrent operation. Due to very high restrictions on memory operations, this model has low performance. | This model is the strictest of all consistency models. The model works on the concept of atomicity. In this model, all the memory accesses are performed atomically that is one memory access from any one processor can be executed at any given time and secondly the whole sequence of memory accesses are executed entirely. They can not be interrupted by any concurrent operation. Due to very high restrictions on memory operations, this model has low performance. | ||
====Sequential | ====Sequential Consistency==== | ||
<ref>http://en.wikipedia.org/wiki/Sequential_consistency</ref> | <ref>http://en.wikipedia.org/wiki/Sequential_consistency</ref> | ||
Line 244: | Line 244: | ||
2) The second technique of performance enhancement depends on speculation access where the execution of a load can be overlapped with the execution of the older load by speculatively assuming that the older load is executed atomically. If the older load doesnot get executed atomically then this younger load is canceled and then it is executed again. This speculative execution is typically only implemented for loads. All stores are issued to the cache atomically only. | 2) The second technique of performance enhancement depends on speculation access where the execution of a load can be overlapped with the execution of the older load by speculatively assuming that the older load is executed atomically. If the older load doesnot get executed atomically then this younger load is canceled and then it is executed again. This speculative execution is typically only implemented for loads. All stores are issued to the cache atomically only. | ||
====Causal | ====Causal Consistency==== | ||
<ref>http://en.wikipedia.org/wiki/Causal_consistency</ref> | <ref>http://en.wikipedia.org/wiki/Causal_consistency</ref> | ||
Line 255: | Line 255: | ||
Here, load operation r3(x) is casually dependent on store w2(x) and load operation r4(x) is casually dependent on store w1(x). | Here, load operation r3(x) is casually dependent on store w2(x) and load operation r4(x) is casually dependent on store w1(x). | ||
====PRAM Consistency==== | |||
<ref>http://en.wikipedia.org/wiki/PRAM_consistency</ref> | |||
PRAM consistency is pipelined random access memory consistency. It is also known as FIFO consistency. This consistency model is even more relaxed than casual consistency model. In this consistency model, all processors see the stores performed by one processor in the same order as they were issued from that processor. But stores performed by different processers may be seen in a different order by different processors. In this consistency model, only the store order needs to be consistent not the load and that is why it is named as pipelined. PRAM consistency is easy to implement. The model simply says that there are no guarantees about the order in which different processors see the stores, except that two or more stores performed by the single processor must arrive in order, as if they were in a pipeline. | |||
INSERT IMAGE HERE | |||
====Cache Consistency Model==== | |||
Cache Consistency is dealt using cache coherence protocols. This model simply requires that all store operations to the same memory location by the processors are performed in some sequential order. Also, the apearance of their order to other processors is same as the order in which they are performed. | |||
====Slow Consistency Model==== | |||
The Slow Consistency Model is weaker than PRAM Consistency Model. This model states that when a load operation is performed on a memory location then the memory returns the latest write operation performed on it. But succeeding loads to the same location may not return stores issued earlier (by the processor that issued the earlier stores) than the first load. | |||
INSERT IMAGE HERE | |||
====Local Consistency Model==== | |||
Local Consistency Model is considered to be the weakest model. The model requires that all the load and store operations by the performed by a processor are apeared to that processor as if they are performed on a single processor system. Although, there is no restriction on how the order of memory operations performed by different processors will apear to different processors. | |||
====Relaxed Consistency Models==== | |||
These models basically loosen up the memory access restrictions of Sequential Consistency model. As these models do not put a lot of restriction on the sequence of the memory access thus their performance is better than Sequential Consistency Model. But the implementation of these models require a lot of complexity in the program. During coding, the programmers have to make sure that their programs obey the rules of the consistency model that the hardware provides. | |||
Relaxation in the memory accesses may allow the order of execution of the instructions in a program different from the programmer’s expectation. Thus to prevent any non-deterministic outcome of the program, safty net is provided to the programmers to implement it in their program to specify strict ordering between a pair of memory accesses. This safty net is provided in terms of fence instruction. Once a fence instruction is implemented in the program then it prohibits all the memory accesses following it until all the memory accesses before it have been completed. Fence instruction is of two types. When the fence is only applied to the stores such that there is a proper ordering of the memory accesses between all the stores before the fence and all following it then such type of fence is called store fence/barrier. On the other side, when the fence is only applied to the loads such that there should be perfect ordering between the loads before the fence apears and the loads after the fence then such a fence is called load fence/barrier. | |||
When a fence is implemented in the program then all the memory accesses younger than fence are flushed out from the pipeline. Firstly all the memory accesses older than fence are completed. After that the processor state is restored to the state prior to the fence instruction and execution is resumed from that point. | |||
=====Processor Consistency Model===== | |||
In Sequential Consistency, on all the memory accesses, a proper ordering is forced that is Load -> Load, Load -> Store, Store -> Store, and Store -> Load. The basic implementation of a Processor Consistency Model is based on relaxing the ordering between an older store and a younger store (Store -> Load). In this model, when a store has not completed, a younger load is allowed to issue to the cache and even complete. The importance of this is that store instructions can be queued in the write buffer and be completed at a later time without the use of load speculation. In the mean time, loads do not require to wait for the older stores to complete and can access the cache and hence they reduce the load latency. In case of PC, when a load is issued to the chache then it is not rolled back even if the illusion of atomicity is broken in contrast to SC where the load is rolled back at once. This result is better performance of PC as compared to SC. PC only relaxes one ordering rule out of four, hence it only affects the correctness of code that has a store followed by a load. If a program is properly synchronized programs, PC provides the same outcome as SC. In a post and wait synchronization in which a processor produces a data value and sets a flag to signal tha availability of the new value. This involves at least two store instructions, which are ordered with respect to each other in PC. Once the comsumer sees the flag high the it performs at least two loads and this ordering is also gurenteed under PC. Thus, post and wait sysnc produces te same outcome in PC as in SC. | |||
INSERT IMAGE HERE | |||
=====Weak Ordering Model===== | |||
<ref>http://en.wikipedia.org/wiki/Weak_consistency</ref> | |||
When a programmer writes a program then he makes sure that the ordering issue is addressed using synchronization primitives. So it could be said that a majority of the programs are properly synchronized. These synchronization primitives can be of the form of barriers, point-to-point synchrnozation, lock etc. Also it can be concluded that if a program is properly synchrnonized , there is no data race that can occur. Data race is defined as simultaneous accesss of a single locations by multiple threads in which at least one of the access is a store. Simulatanous loads do not chance the outcome of the loads hence they cannot produce data race. Simulatanous stores may overwirte each other hence they can induce data race. A simulatanoues load and store also may cause the load to return different values. The implication of the lack of data race in a properly synchronizaed program is that the ordering of memory accesses can be relaxed except at synchronization points. This concept is implemented in Weak Ordering Consistency Model. The WO model uses 2 assumptions: | |||
1) Programs are properly synchronized and | |||
2) Programmers correctly express to the hardware which loads and stores act as syncrhonization accesses. | |||
Based on the assumptions, we can define the correct behaviour of a synchronization access: | |||
1) Before a synchronization access can issue, all prior loads, stores and synchronization accesses must have completed and | |||
2) All loads, stores. And synchronization accesses following it must not have issued. | |||
======Basic Implementation of WO ====== | |||
When a synchronization access is encountered at the processor pipeline, first of all, all the memory accesses following this synchronization point are flushed out of the processor pipeline. Then this synchronization accees itself is made to halt until all the memory accesses before this synchronization point are completed. All the loads have obtained their value and all the stores have been propagated their values. | |||
WO is more relaxed than PC because a WO compiler can re-order the memory accesses and it just have to make sure that they do not cross the synchronization point. In PC only Store Load access is relaxed. WO works well if the critical section is big containing more memory accesses to be executed. If the critical section is small then there is very less opportunities to reorder the memory accesses as there are less number of them. So when critical section is small then PC outperforms WO. WO is more relaxed but when program complexity is concerned then PC is better than WO. | |||
INSERT IMAGE HERE | |||
INSERT CODE HERE | |||
=====Release Consistency Model===== | |||
<ref>http://en.wikipedia.org/wiki/Release_consistency</ref> | |||
The Release Consistency model implementation is based on two different types of syncrhonization accesses: | |||
Acquire syncrhonization (lock): which ensures that no younger load/store executes before the acquire is complete. Thus it prevents upward migration of memory accesses but not downward migration. | |||
Release syncronization (unlock): which ensures that all older loads/stores complete before the release is issued. This it prevents downward migration of memory accesses but no upward migration. | |||
It is also made sure in this model that acquires and releases must execute atomically with respect to one another. This implies that they must not appear to have overlapped their execution with respect of one another. This model requires all syncronization accesses in a program to be indetified correctly and completely, so that the hardware can ensure correct execution of properly synchronized program. Programming complexity is higher in RC than in WO. | |||
======Basic Implementation of RC ====== | |||
The release synchronization must prevent downward migration, so when a release synchronization access is encountered in the processor pipeline, the release access is halted until all prior memory accesses have completed. An acquire syncrhonization must prevent upward migration. When an acquire synchronization access is encountered in the processor pipeline, all instructions younger than it (including all loads and stores) are cancled and re-executed after the acquire syncrhonization completes. Similar to WO, RC allows the compiler to freely recorder loads and stores excep that they cannot migratre upward past as acquire synchronization and cannot migrate downward past a release synchronization . However, this flexibility and perfornamce advantage of RC results in higher complexity of the program. | |||
INSERT IMAGE HERE | |||
INSERT IMAGE HERE | |||
=====Lazy Release Consistency Model===== | |||
The Lazy Release Consistency model is a further optimization of the Release Consistency model (RC) in which it is considered that the thread that executes an acquire synchronization does not need values written by another thread until the acquire synchronization is completed. When a store is executed in a critical section then before the unlock is executed it is made sure that the this memory access is propagated fully to the other caches. Then the unlock operation is executed which involves another store where the value of lockvar is changed to 0. This value is again propagated fully to other caches and then after that the lock operation is executed. It can be concluded that the value of the store in the first critical section is not required until the lock operation for the second critical section is executed. So it can be said that the propagation of the values written prior to the release synchronization can be delayed until the release synchronization is complete and then both the values wiritten in the critical section and on release synchronization can be propagated togather to the other caches. This model is beneficial in the cases where there is a little bandwidth available between the processors or in a system where there are high overheads due to the propagation of small amount of data or infrequent propagattion of large amount of data. | |||
INSERT IMAGE HERE | |||
====Other Consistency Models==== | |||
=====Delta Consistency Model===== | |||
<ref>http://en.wikipedia.org/wiki/Delta_consistency</ref> | |||
This model is a core functionality that gives content providers “freshness guarantee” by ensuring that updates will propagate through the system and all replicas will be consistent after a fixed time period. | |||
=====Entry Consistency Model===== | |||
<ref>http://en.wikipedia.org/wiki/Entry_consistency</ref> | |||
This model requires the programmer to use acquire and release at the start and end of each critical section, respectively. Entry consistency requires each ordinary shared variable to be associated with some synchronization variable such as a lock or barrier. If it is desired that elements of an array be accessed independently in parallel, then different array elements must be associated with different locks. | |||
=====Eventual Consistency Model===== | |||
<ref>http://en.wikipedia.org/wiki/Eventual_consistency</ref> | |||
This model states that given a sufficiently long period of time over which no changes are sent, all updates can be expected to propagate eventually through the system and the replicas will be consistent. | |||
=====Fork Consistency Model===== | |||
<ref>http://en.wikipedia.org/wiki/Fork_consistency</ref> | |||
In this model, if an untrusted server hides the modifications of one writer to another, the latter will not be shown any future modifications of the former, effectively forking the views of them. This is the strongest consistency model that can be achieved with an untrusted server. | |||
=====One-Copy Consistency Model===== | |||
<ref>http://en.wikipedia.org/wiki/One-copy_serializability</ref> | |||
This model is the same as sequential consistency, as though there is only one copy of the file, thus, access requests are fully serialized. | |||
=====One-Copy Consistency Model===== | |||
<ref>http://en.wikipedia.org/wiki/One-copy_serializability</ref> | |||
This model is the same as sequential consistency, as though there is only one copy of the file, thus, access requests are fully serialized. | |||
=====Vector-Field Consistency Model===== | |||
<ref>http://en.wikipedia.org/wiki/Vector-field_consistency</ref> | |||
This is a consistency model for replicated data. It is designed to allow bounded divergence of object replicas. In this model replica consistency is selectively and dynamically strengthened or weakened based on the on-going game state and simultaneously it manages: how the consistency degree is changed throughout the execution; and how the consistency requirements are specified. | |||
== Memory Coherence and Shared Virtual Memory == | == Memory Coherence and Shared Virtual Memory == |
Revision as of 09:30, 17 March 2015
Previous Page: http://wiki.expertiza.ncsu.edu/index.php/CSC_456_Spring_2012/ch7_AA
Current Page: http://wiki.expertiza.ncsu.edu/index.php/CSC/ECE_506_Spring_2013/7a_bs
Introduction
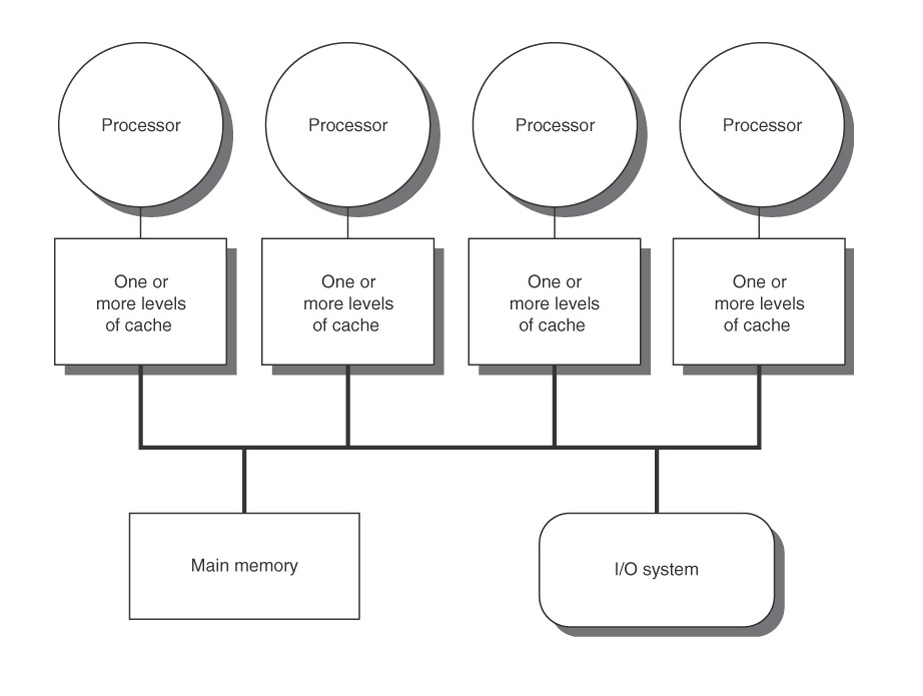
A cache is considered coherent if its read operations always return the most recently written values at the same address. In a system with a single processor (single core), maintaining cache coherence is simple and easy, but in a multiprocessor system, it is not as simple. Data can be present in any processor's cache, and protocol needs to ensure that the data is same in every cache. If it cannot ensure that all the caches are the same, then it needs to flag a cache line to indicate that it is not updated.
In the figure shown here, there is a 4 processor shared memory system where each processor has its own cache. Suppose processor P1 reads memory location M1 and stores it in its local cache. Then, processor P2 also reads from M1 and stores its own local cache. Now, if P1 changes value of M1, there will be two copies of same data residing in different caches, but the one in P1's cache will be different. The problem arises when P2 operates on M1, and uses the stale value of M1 that was stored in its cache.
Cache Coherence
One may think that cache write policy can provide cache coherence, but it is not true. Cache write policy only controls how a change in value of a cache is propagated to a lower level cache or main memory. It is not responsible for propagating changes to other caches.
In order to understand what this means, we need to first understand what cache coherence is and compare it with the purpose of a cache write policy.
Cache coherence refers to the consistency of data stored in local caches of a shared resource.
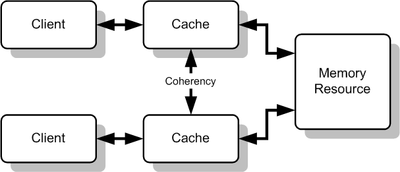
In a shared memory multiprocessor system, each processor may have a separate cache. Hence, in such conditions, it is possible to have multiple copies of the instruction in each cache; one copy in the main memory and one in each cache memory. Here, when one copy of an operand is changed, the other copies of the operand must be changed also. Thus, cache coherence is the discipline that ensures that changes in the values of shared operands are propagated throughout the system in a timely fashion. Examples of cache coherence protocols include MSI, MOESI, MESI etc.
A Cache write policy on the other hand refers to the write policy by which the cache handles writes to the lower level caches or the main memory. The timing of this write is controlled by what is known as the write policy.
There are two basic writing policies<ref>http://en.wikipedia.org/wiki/Cache_(computing)</ref>:
• Write-through – here, the write is done synchronously, both to the cache and to the backing store.i.e Every time the processor updates a cached memory location, both the cache and the underlying memory location is updated.
• Write-back (or Write-behind or copy back) – here, writing is initially done only to the cache. The write to the backing store is postponed until the cache blocks containing the data are about to be modified/replaced by new content<ref>http://www.pcguide.com/ref/mbsys/cache/funcWrite-c.html</ref>.
Hence, we see that it is not the responsibility of the cache write policy to propagate changes to others caches, but only to the lower level memory. It is the responsibility of the Coherance protocols followed to implement this.
Cache coherence<ref>http://en.wikipedia.org/wiki/Cache_coherency</ref> solutions are mainly classified as software-based or hardware-based solutions. Software-based solutions can be implemented using compiler-based or run-time system support. In addition, some of them can also be done with hardware assistance. Hardware-based solutions can also be implemented in many different ways. For example, one approach could be done by having each cache controller listen for traffic on the bus that would invalidate its cache line. Alternatively, the solution could use directories which track which cache blocks most recently accessed a particular cache block. Another variation of implementation is write-through versus write-back; the former writes to main memory whenever a cache is written to, while the latter waits to update main memory until the cache block is evicted.
The main concern in the case of software-based solutions is that perfect information is needed at all times when memory aliasing and explicit parallelism are required. So, the focus is more on improving hardware-based solutions, making them more common. Studies have shown that different snoop-based cache coherence schemes are more strongly sensitive toward write-policy than the specific coherence protocol. Write-back schemes are more efficient despite the increased hardware complexity involved in cache coherence support.
Hardware-based cache-coherence protocols, though more competitive in terms of performance with respect to basic architectures with no hardware support, incur significant power cost as coherence traffic grows. Thus, as power constraints become tighter and the degree of multiprocessing increases, viability of hardware-based solutions becomes doubtful.
Protocols
Whenever a processor changes a word in its cache, all other caches holding the value must be notified. In order to keep their own values consistent with the freshly written cache, the cache's could be either updated or invalidated. In the update method, if variable 'x' is modified by core 1, core 1 has to send the updated value of 'x' onto the inter-core bus. Each cache listens to the inter-core bus and if a cache sees a variable on the bus which it has a copy of, it will read the updated value. This ensures that all caches have the most up-to-date value of the variable.
In case of invalidation, an invalidation message is sent onto the inter-core bus when a variable is changed. The other caches will read this invalidation signal; if its core attempts to access that variable, it will result in a cache miss and the variable will be read from main memory.
The update method results in significant amount of traffic on the inter-core bus as the update signal is sent onto the bus every time the variable is updated. The invalidation method only requires that an invalidation signal be sent the first time a variable is altered; this is why the invalidation method is the preferred method.
Invalidate Coherence Protocols
- MSI
MSI stands for Modified, Shared, and Invalid, which is based on the three states that a line of cache can be in. The Modified state means that a variable in the cache has been modified and therefore has a different value than that found in main memory; the cache is responsible for writing the variable back to main memory. The Shared state means that the variable exists in at least one cache and is not modified; the cache can evict the variable without writing it back to the main memory. The Invalid state means that the value of the variable has been modified by another cache and is invalid; the cache must read a new value from main memory (or another cache).
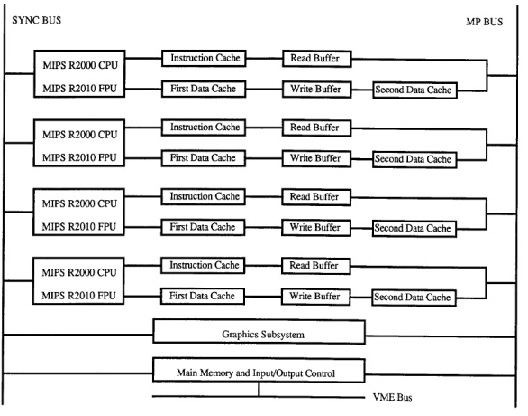
- MESI
MESI stands for Modified, Exclusive, Shared, and Invalid. The Modified and Invalid states are the same for this protocol as they are for the MSI protocol. This protocol introduces a new state; the Exclusive state. The Exclusive state means that the variable is only in this cache and its value matches the value within the main memory. This now means that the Shared state indicates that the variable is contained in more than one cache.
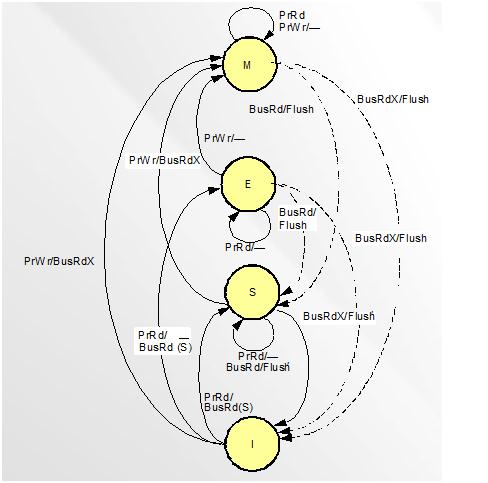
- MOSI
The MOSI protocol is identical to the MSI protocol except that it adds an Owned state. The Owned state means that the processor "Owns" the variable and will provide the current value to other caches when requested (or at least it will decide if it will provide it when asked). This is useful because another cache will not have to read the value from main memory and will receive it from the Owning cache much, much, faster.
- MOESI<ref>http://en.wikipedia.org/wiki/MOESI_protocol</ref>
MOESI is a full cache coherency protocol that encompasses all of the possible states commonly used in other protocols. In addition to the four common MESI protocol states, there is a fifth "Owned" state representing data that is both modified and shared. This avoids the need to write modified data back to main memory before sharing it. While the data must still be written back eventually, the write-back may be deferred. In order for this to be possible, direct cache-to-cache transfers of data must be possible, so a cache with the data in the modified state can supply that data to another reader without transferring it to memory.
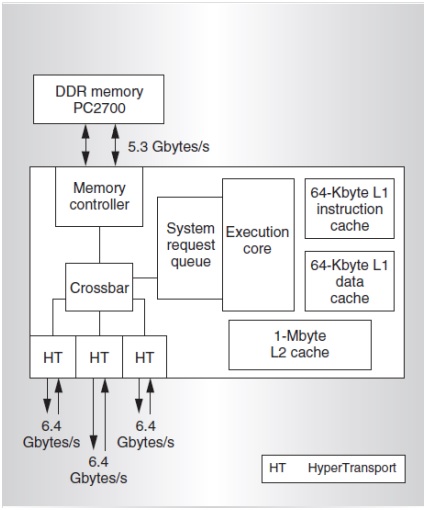
- MESIF<ref>http://en.wikipedia.org/wiki/MESIF_protocol</ref>
The MESIF protocol is a cache coherency and memory coherence protocol developed by Intel for cache coherent non-uniform memory architectures.The protocol consists of five states, Modified (M), Exclusive (E), Shared (S), Invalid (I) and Forward (F). The 'M', 'E', 'S' and 'I' states are the same as in the MESI protocol. The 'F' state is a specialized form of the 'S' state, and indicates that a cache should act as a designated responder for any requests for the given line. The protocol ensures that, if any cache holds a line in the S state, at most one (other) cache holds it in the F state. In a system of caches employing the MESI protocol, a cache line request that is received by multiple caches holding a line in the S state will be serviced inefficiently. It may either be satisfied from (slow) main memory, or all the sharing caches could respond, bombarding the requestor with redundant responses. In a system of caches employing the MESIF protocol, a cache line request will be responded to only by the cache holding the line in the F state.This allows the requestor to receive a copy at cache-to-cache speeds, while allowing the use of as few multicast packets as the network topology will allow.
Update Coherence Protocols
Update based cache coherence protocols directly update all the cache values in the system.This is different from invalidation based protocols as it achieves write propagation without having to invalidate. Hence this reduces coherence misses and also bandwidth usage.The two update based protocols- Dragon Protocol and Firefly Protocol are discussed below.
- Dragon Protocol
The Dragon Protocol is an update-based coherence protocol. It reduces bandwidth as it updates the specific words instead of entire block within the cache.Write allocate and write update policies are used by the caches. The Dragon Protocol consists of four states (Modified (M), Exclusive (E), Shared Clean (Sc), and Shared Modified (Sm)) and there is no invalidation state,because every block present in the cache is valid.
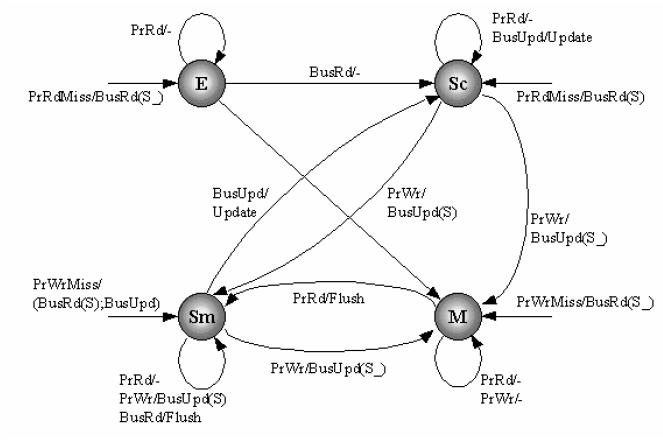
- Firefly protocol
This is another example of update-based coherence cache protocols.Unlike the Dragon Protocol, the caches use a write-through policy and hence writes all changes to memory.This protocol uses a special bus technique called SharedLine to allow quick detection to copies of the block in other caches. It is similar to the COPIES_EXIST(C) and !COPIES_EXIST. In the firefly protocol , there is no invalidation state just like the dragon protocol as the cache blocks are never invalidated.
Hybrid Protocols<ref>http://wiki.expertiza.ncsu.edu/index.php/User:Stchen#Introduction_to_Update_and_Adaptive_Coherence_Protocols_on_Real_Architectures</ref>
Even though there are clear advantages to using either update protocols or invalidate protocols, there are still disadvantages for each. In a write invalidate protocol, any update/write operation in a processor invalidates the shared cache blocks of other processors. This will force the other caches to do read requests such that the new data is retrieved. This tends to cause a high bus bandwidth and can be especially bad if there is few processors that frequently update the cache. Fortunately, write update protocols mitigate this issue. It will update all other caches at the same time it propagates an update itself. Unfortunately, this creates a different problem, there will sometime be unnecessary update to the cache on the other processors. This tends to increase conflict and capacity cache misses.
Hybrid protocols help to mitigate these problems by decreasing some high bus traffic as well as some unnecessary updates. But,this is possible based on how the adaptive algorithm switches between write-invalidate and write-update.
- Competitive update cache coherence protocol<ref>H. Nilsson, P. Stenström
An adaptive update-based cache coherence protocol for reduction of miss rate and traffic Proc. Parallel Architectures and Languages Europe (PARLE) Conf., Lecture Notes in Computer Science, Athens, Greece, 817, Springer-Verlag, Berlin (Jul. 1994)</ref> This protocol is a hybrid between write-invalidate and write-update protocols and for a wide range of applications it outperforms write invalidate protocols under relaxed memory consistency models because of a lower miss rate. In this protocol,a copy of the block is updated instead of being invalidated at the first write by another processor. If the local processor does not access the copy,it is invalidated after a number of global updates determined by a competitive threshold. As a result, only those copies regularly accessed are updated. In competitive-update protocols coherence is maintained by update messages rather than invalidation messages. Upon a write request, update messages are sent to all caches sharing the same memory block. In contrast to write-update protocols, a competitive threshold, C, is used to locally invalidate copies that are not accessed by the local processor between a number of updates; i.e., when a copy has been updated C times it is invalidated and the update messages to it cease. Thus, the network traffic is reduced compared to a write-update protocol since only those copies regularly accessed are updated.
- Cachet<ref>http://csg.csail.mit.edu/pubs/memos/Memo-414/memo-414.pdf</ref>
Cachet provides wide scope for adapting to changing program behaviors.It is especially suitable for large Distributed Shared Memory (DSM) systems, and applicable to a wide variety of programmer-centric memory models.Cachet allows write operations to be performed without the exclusive ownership. This not only alleviates potential cache thrashing due to false sharing, but also reduces the average latency of write operations.
Memory Consistency
Memory consistency deals with the ordering of all memory operations/accesses (loads and stores) to different memory locations. It can be summarized as the rule which must be enforced among all loads and stores to different locations in a shared memory by different processors. It can also be present in systems without caches.
Memory Consistency Model
In order to maintain memory consistency in a multiprocessor system when a program is executed on it, a model is required to specify the behavior of how the memory has to be accessed or to provide an agreement between the memory implementation and the program which is going to utilize this memory. This model is called Memory Consistency Model and It is used to define the ordering in which all loads and stores must be performed, both relative to each other in the same program and to other loads and stores by another program on another processor. The programmer before writing the code must have knowledge of the Memory Consistency Model used by the hardware in order to ensure program correctness. The code below from Solihin<ref>Yan Solihin. "Fundamentals of Parallel Computer Architecture: Multichip and Multicore Systems." Solihin Publishing & Consulting LLC, 2009.</ref> shows an example of possible inconsistency:
P0 P1 S1 : datum = 5 S3 : while(!datumIsReady) {} S2 : datumIsReady = 1 S4 : print datum
In this example, P0 generates sets the values of datum and datumIsReady. By setting datumIsReady to 1, this signals P1 that datum is now ready. P1 spins in the while loop waiting for this flag to become 1 and then prints datum. In this example an in similar cases, it is important that the compiler understand what the programmer intended so that the program order is preserved. Within a uni-processor, this problem can be solved by declaring which variables must be synchronized. For instance when using C, this can be accomplished by declaring variables that may be susceptible to inconsistency as volatile.
When a programmer writes a code, then he implicitly expects that the order of all memory accesses (loads and stores ) coming out of a pocessor will be performed in the program order and each memory access will be performed atomically. The code below from Solihin<ref>Yan Solihin. "Fundamentals of Parallel Computer Architecture: Multichip and Multicore Systems." Solihin Publishing & Consulting LLC, 2009.</ref> shows another example of possible inconsistency:
P0 P1 P3 S1 : x = 5 S3 : while(!xReady) {} S6: while(!xyReady) S2 : xReady = 1 S4 : y = x + 4 S7: z = x * y xyReady = 1
In the above example, initially, the values of x, y, z, xReady and xyReady are zero. If we consider that the program order to S1 S2 S3 S4 S5 S6 S7.
So as per the code, firstly P0 executes its statements S1 and S2 in order and sets the value of x as 5 and then xReady as 1. After that, P1 executes its statements in order of S3 then S4 then S5. P1 reads the value of xReady and comes out of the loop and then reads the value of latest x (which is 5) to calculate the value of y. Once it is calculated then the variable xyReady is set to 1. P3 then checks the value of the variable xyReady and comes out of the loop. Then it reads the latest value of x and y (which is 5 and 9) and find the value of z. The programmer expects that the value of z should be 45 (5*9) due to the atomicity of the execution of the instructions.
Now, consider the following scenario. P0 executes S1, which writes a value of 5 to x. This write is then propagated to P1 and P2, however it reaches P1 faster that P2. Then, P0 performs a write to xReady, which is also propagated to P1. P1 now sees the new values of xReady and x. So P1 excutes its S4 statement, assigning a value of 9 to y, and then sets xyReady to 1which is propagated to P2. P2 then gets out of its loop and reads the value of x and y. Since the value of x from P0 has yet not reached P2, P2 reads a fresh value of y but the stale value of x. Thus the output of the program is un-expected which in this case is z = 0.
INSERT PICTURE HERE
From the first and second example we can understand that the implicit expectation of the programmer is:
1) Memmory accesses performed by a single processor should be occured in program order and
2) Each of them should be performed atomically. Such an expectation was formally defined as the Sequential Consistency which we are going to Describe first.
Memory Semantics in Uniprocessor Systems
Uniprocessor languages use simple sequential semantics for memory operations, which allow the programmer to assume that all memory operations will occur one at a time in the sequential order specified by the program. Thus, the programmer can expect a read to return the value of the most recent write to the location according to sequential program order. It is sufficient to only maintain uniprocessor data and control dependences. The compiler and hardware can freely reorder operations to different locations if the uniprocessor data and control dependences are respected. This enables compiler optimizations such as register allocation, code motion, and loop transformations, and hardware optimizations, such as pipelining, multiple issue, write buffer bypassing and forwarding, and lockup-free caches, all of which lead to overlapping and reordering of memory operations.<ref>Sarita V. Adve. Kourosh Gharachorloo. "Shared Memory. Consistency Models: A Tutorial." Digital Western Research Laboratory 250 University Avenue Palo Alto. <http://www.hpl.hp.com/techreports/Compaq-DEC/WRL-95-7.pdf</ref>
Memory Semantics in Multiprocessor Systems
Unfortunately, memory consistency is not as straight-forward on multiprocessors. With regard to the example above, instead of each process running on a separate thread, each process runs on a separate processor. With multiple processors, more problems arise with respect to order of execution. While one process might execute instructions in program order, other processes may not recognize the executions in the same order due to delays in communication. How processors handle this problem depends on the chosen consistency model<ref>http://www-vs.informatik.uni-ulm.de/teach/ss05/dsm/arizona.pdf</ref>.
Types of Memory Consistency Models
INSERT MCM_TREE HERE
The tree represents most of the memory consistency models. There are some other memory consistency models as well. These are:
1) Delta Consistency Model
2) Entry Consistency Model
3) Eventual Consistency Model
4) Fork Consistency Model
5) One-Copy Serializability
6) Vector-Field Consistency Model
Following are the detailed explanation of consistency models<ref>http://en.wikipedia.org/wiki/Consistency_model</ref>:
Atomic or Strict Consistency
<ref>http://en.wikipedia.org/wiki/Linearizability</ref> This model is the strictest of all consistency models. The model works on the concept of atomicity. In this model, all the memory accesses are performed atomically that is one memory access from any one processor can be executed at any given time and secondly the whole sequence of memory accesses are executed entirely. They can not be interrupted by any concurrent operation. Due to very high restrictions on memory operations, this model has low performance.
Sequential Consistency
<ref>http://en.wikipedia.org/wiki/Sequential_consistency</ref> The most commonly assumed memory consistency model for shared memory multiprocessors is Sequential Consistency Model. This model is similar to Atomic Consistency but it contains the possiblity of optimizations to improve the performance. Sequential Consistency was formally defined by Lamport as follows:
“A multiprocessor system is sequentially consistent if the result of any execution is the same as if the operations of all the processors were executed in some sequential order, and the operations of each individual processor appear in this sequence in the order specified by its program.”
There are two main aspects to sequential consistency:
(1) Maintenance of the program order among memory operations from individual processors
(2) Maintenance of a single sequential order among memory operations from all processors
The second aspect makes it appear as if one memory operation executes atomically or instantaneouslywith respect to other memory operations.
INSERT IMAGE HERE
Basic Implementation of Sequential Consistency
The basic implementation of SC involves execution of one memory access at a time without overlapping it with another memory access. Thus it is required to understand the starting and ending point of a particular memory access. Memory accesses are performed when either there is a load or a store operation: Let us consider a load operation,
Step 1- computation of effective address in a functional unit.
Step 2- cache access to the effective address issued by memory hierarchy.
Step 3- fetching the data value associated with the address.
Step 4- returning the value to the destination register of the load instruction.
Here, it is clear that step 1 and step 4 are not affected by other memory accesses. Effective address is computed in the functional unit which involves computation not access. Similarly the final value to be loaded in the destination register has already been fetched from the cache so it does not invlove any further memory access. Thus it can be concluded that the actual load (which involves memory access) starts from step 2 and ends on step 3. These two steps should be performed atomically.
Let us consider store operations:
Step 1- computation of effective address in a functional unit.
Step 2- the store is consigned by copying the destination address and the latest value to be written to a write buffer.
Step 3- the store value in the write buffer is released to the cache.
Step 4- the store is considered complete only when this new value is fully propagated to the other processors.
As the store invloves multiple cache values thus effectively the store starts when the destination address is issued and the latest value is stored in the write buffer in step 2 till the step 4 where the store is fully propagated to other caches. So these steps should be performed atomically. It can be concluded that the basic implementation of SC inflicts a lot of restriction on how the intructions within a program have to executed and thus it affects the performance. So in order to improve the performance of SC, few optimizations in the model are possible . INSERT IMAGE HERE
Techniques to improve Sequential Consistency Performance
It can be concluded that the performance of an SC implementation can be improved if the execution of memory accesses is made faster and when it is allowed for the memory accesses to be overlapped with respect to one another.
1) The first performance enhancement technique avoids the overlapping of execution of loads and stores but involves overlapping of the data fetches that are generated by these loads and stores.
2) The second technique of performance enhancement depends on speculation access where the execution of a load can be overlapped with the execution of the older load by speculatively assuming that the older load is executed atomically. If the older load doesnot get executed atomically then this younger load is canceled and then it is executed again. This speculative execution is typically only implemented for loads. All stores are issued to the cache atomically only.
Causal Consistency
<ref>http://en.wikipedia.org/wiki/Causal_consistency</ref> Casual Consistency Model is a weaker Model of Sequential Consistency Model. Here, a differentiation is made between events that are potentially causally connected with each other and those that are not. A system provides Causal Consistency if memory operations that potentially are causally related are seen by every processor of the system in the same order. Operations that are not causally related are said to be concurrent. Concurrent writes (i.e. ones that are not causally related) may be seen in different order by different processors but Condition-writes (i.e. ones that are potentially causally related) must be seen by all processes in the same order.
When a store operation follows a load operation performed by a processor then the load and store are considered casually connected. Similarly, when a load operations follows a store operation then it is also considered casually connected. Also, even two stores operations performed by the same processor are defined to be causally ordered, in the order they were performed.
INSERT IMAGE HERE
Here, load operation r3(x) is casually dependent on store w2(x) and load operation r4(x) is casually dependent on store w1(x).
PRAM Consistency
<ref>http://en.wikipedia.org/wiki/PRAM_consistency</ref> PRAM consistency is pipelined random access memory consistency. It is also known as FIFO consistency. This consistency model is even more relaxed than casual consistency model. In this consistency model, all processors see the stores performed by one processor in the same order as they were issued from that processor. But stores performed by different processers may be seen in a different order by different processors. In this consistency model, only the store order needs to be consistent not the load and that is why it is named as pipelined. PRAM consistency is easy to implement. The model simply says that there are no guarantees about the order in which different processors see the stores, except that two or more stores performed by the single processor must arrive in order, as if they were in a pipeline.
INSERT IMAGE HERE
Cache Consistency Model
Cache Consistency is dealt using cache coherence protocols. This model simply requires that all store operations to the same memory location by the processors are performed in some sequential order. Also, the apearance of their order to other processors is same as the order in which they are performed.
Slow Consistency Model
The Slow Consistency Model is weaker than PRAM Consistency Model. This model states that when a load operation is performed on a memory location then the memory returns the latest write operation performed on it. But succeeding loads to the same location may not return stores issued earlier (by the processor that issued the earlier stores) than the first load.
INSERT IMAGE HERE
Local Consistency Model
Local Consistency Model is considered to be the weakest model. The model requires that all the load and store operations by the performed by a processor are apeared to that processor as if they are performed on a single processor system. Although, there is no restriction on how the order of memory operations performed by different processors will apear to different processors.
Relaxed Consistency Models
These models basically loosen up the memory access restrictions of Sequential Consistency model. As these models do not put a lot of restriction on the sequence of the memory access thus their performance is better than Sequential Consistency Model. But the implementation of these models require a lot of complexity in the program. During coding, the programmers have to make sure that their programs obey the rules of the consistency model that the hardware provides.
Relaxation in the memory accesses may allow the order of execution of the instructions in a program different from the programmer’s expectation. Thus to prevent any non-deterministic outcome of the program, safty net is provided to the programmers to implement it in their program to specify strict ordering between a pair of memory accesses. This safty net is provided in terms of fence instruction. Once a fence instruction is implemented in the program then it prohibits all the memory accesses following it until all the memory accesses before it have been completed. Fence instruction is of two types. When the fence is only applied to the stores such that there is a proper ordering of the memory accesses between all the stores before the fence and all following it then such type of fence is called store fence/barrier. On the other side, when the fence is only applied to the loads such that there should be perfect ordering between the loads before the fence apears and the loads after the fence then such a fence is called load fence/barrier.
When a fence is implemented in the program then all the memory accesses younger than fence are flushed out from the pipeline. Firstly all the memory accesses older than fence are completed. After that the processor state is restored to the state prior to the fence instruction and execution is resumed from that point.
Processor Consistency Model
In Sequential Consistency, on all the memory accesses, a proper ordering is forced that is Load -> Load, Load -> Store, Store -> Store, and Store -> Load. The basic implementation of a Processor Consistency Model is based on relaxing the ordering between an older store and a younger store (Store -> Load). In this model, when a store has not completed, a younger load is allowed to issue to the cache and even complete. The importance of this is that store instructions can be queued in the write buffer and be completed at a later time without the use of load speculation. In the mean time, loads do not require to wait for the older stores to complete and can access the cache and hence they reduce the load latency. In case of PC, when a load is issued to the chache then it is not rolled back even if the illusion of atomicity is broken in contrast to SC where the load is rolled back at once. This result is better performance of PC as compared to SC. PC only relaxes one ordering rule out of four, hence it only affects the correctness of code that has a store followed by a load. If a program is properly synchronized programs, PC provides the same outcome as SC. In a post and wait synchronization in which a processor produces a data value and sets a flag to signal tha availability of the new value. This involves at least two store instructions, which are ordered with respect to each other in PC. Once the comsumer sees the flag high the it performs at least two loads and this ordering is also gurenteed under PC. Thus, post and wait sysnc produces te same outcome in PC as in SC.
INSERT IMAGE HERE
Weak Ordering Model
<ref>http://en.wikipedia.org/wiki/Weak_consistency</ref> When a programmer writes a program then he makes sure that the ordering issue is addressed using synchronization primitives. So it could be said that a majority of the programs are properly synchronized. These synchronization primitives can be of the form of barriers, point-to-point synchrnozation, lock etc. Also it can be concluded that if a program is properly synchrnonized , there is no data race that can occur. Data race is defined as simultaneous accesss of a single locations by multiple threads in which at least one of the access is a store. Simulatanous loads do not chance the outcome of the loads hence they cannot produce data race. Simulatanous stores may overwirte each other hence they can induce data race. A simulatanoues load and store also may cause the load to return different values. The implication of the lack of data race in a properly synchronizaed program is that the ordering of memory accesses can be relaxed except at synchronization points. This concept is implemented in Weak Ordering Consistency Model. The WO model uses 2 assumptions:
1) Programs are properly synchronized and
2) Programmers correctly express to the hardware which loads and stores act as syncrhonization accesses.
Based on the assumptions, we can define the correct behaviour of a synchronization access:
1) Before a synchronization access can issue, all prior loads, stores and synchronization accesses must have completed and
2) All loads, stores. And synchronization accesses following it must not have issued.
Basic Implementation of WO
When a synchronization access is encountered at the processor pipeline, first of all, all the memory accesses following this synchronization point are flushed out of the processor pipeline. Then this synchronization accees itself is made to halt until all the memory accesses before this synchronization point are completed. All the loads have obtained their value and all the stores have been propagated their values. WO is more relaxed than PC because a WO compiler can re-order the memory accesses and it just have to make sure that they do not cross the synchronization point. In PC only Store Load access is relaxed. WO works well if the critical section is big containing more memory accesses to be executed. If the critical section is small then there is very less opportunities to reorder the memory accesses as there are less number of them. So when critical section is small then PC outperforms WO. WO is more relaxed but when program complexity is concerned then PC is better than WO.
INSERT IMAGE HERE
INSERT CODE HERE
Release Consistency Model
<ref>http://en.wikipedia.org/wiki/Release_consistency</ref> The Release Consistency model implementation is based on two different types of syncrhonization accesses:
Acquire syncrhonization (lock): which ensures that no younger load/store executes before the acquire is complete. Thus it prevents upward migration of memory accesses but not downward migration.
Release syncronization (unlock): which ensures that all older loads/stores complete before the release is issued. This it prevents downward migration of memory accesses but no upward migration.
It is also made sure in this model that acquires and releases must execute atomically with respect to one another. This implies that they must not appear to have overlapped their execution with respect of one another. This model requires all syncronization accesses in a program to be indetified correctly and completely, so that the hardware can ensure correct execution of properly synchronized program. Programming complexity is higher in RC than in WO.
Basic Implementation of RC
The release synchronization must prevent downward migration, so when a release synchronization access is encountered in the processor pipeline, the release access is halted until all prior memory accesses have completed. An acquire syncrhonization must prevent upward migration. When an acquire synchronization access is encountered in the processor pipeline, all instructions younger than it (including all loads and stores) are cancled and re-executed after the acquire syncrhonization completes. Similar to WO, RC allows the compiler to freely recorder loads and stores excep that they cannot migratre upward past as acquire synchronization and cannot migrate downward past a release synchronization . However, this flexibility and perfornamce advantage of RC results in higher complexity of the program.
INSERT IMAGE HERE
INSERT IMAGE HERE
Lazy Release Consistency Model
The Lazy Release Consistency model is a further optimization of the Release Consistency model (RC) in which it is considered that the thread that executes an acquire synchronization does not need values written by another thread until the acquire synchronization is completed. When a store is executed in a critical section then before the unlock is executed it is made sure that the this memory access is propagated fully to the other caches. Then the unlock operation is executed which involves another store where the value of lockvar is changed to 0. This value is again propagated fully to other caches and then after that the lock operation is executed. It can be concluded that the value of the store in the first critical section is not required until the lock operation for the second critical section is executed. So it can be said that the propagation of the values written prior to the release synchronization can be delayed until the release synchronization is complete and then both the values wiritten in the critical section and on release synchronization can be propagated togather to the other caches. This model is beneficial in the cases where there is a little bandwidth available between the processors or in a system where there are high overheads due to the propagation of small amount of data or infrequent propagattion of large amount of data.
INSERT IMAGE HERE
Other Consistency Models
Delta Consistency Model
<ref>http://en.wikipedia.org/wiki/Delta_consistency</ref> This model is a core functionality that gives content providers “freshness guarantee” by ensuring that updates will propagate through the system and all replicas will be consistent after a fixed time period.
Entry Consistency Model
<ref>http://en.wikipedia.org/wiki/Entry_consistency</ref> This model requires the programmer to use acquire and release at the start and end of each critical section, respectively. Entry consistency requires each ordinary shared variable to be associated with some synchronization variable such as a lock or barrier. If it is desired that elements of an array be accessed independently in parallel, then different array elements must be associated with different locks.
Eventual Consistency Model
<ref>http://en.wikipedia.org/wiki/Eventual_consistency</ref> This model states that given a sufficiently long period of time over which no changes are sent, all updates can be expected to propagate eventually through the system and the replicas will be consistent.
Fork Consistency Model
<ref>http://en.wikipedia.org/wiki/Fork_consistency</ref> In this model, if an untrusted server hides the modifications of one writer to another, the latter will not be shown any future modifications of the former, effectively forking the views of them. This is the strongest consistency model that can be achieved with an untrusted server.
One-Copy Consistency Model
<ref>http://en.wikipedia.org/wiki/One-copy_serializability</ref> This model is the same as sequential consistency, as though there is only one copy of the file, thus, access requests are fully serialized.
One-Copy Consistency Model
<ref>http://en.wikipedia.org/wiki/One-copy_serializability</ref> This model is the same as sequential consistency, as though there is only one copy of the file, thus, access requests are fully serialized.
Vector-Field Consistency Model
<ref>http://en.wikipedia.org/wiki/Vector-field_consistency</ref> This is a consistency model for replicated data. It is designed to allow bounded divergence of object replicas. In this model replica consistency is selectively and dynamically strengthened or weakened based on the on-going game state and simultaneously it manages: how the consistency degree is changed throughout the execution; and how the consistency requirements are specified.
The memory coherence problem in a shared virtual memory system and in multicache systems are different. In a multicache multiprocessor, there are processors sharing a physical memory through their private caches. The relatively small size of a cache and the fast bus connection to the shared memory, enables using a sophisticated coherence protocol for the multicache hardware such that the time delay of conflicting writes to a memory location is small.<ref>Kai Li, and Paul Hudak. "Memory coherence in shared virtual memory systems". Published in Journal ACM Transactions on Computer Systems (TOCS), Volume 7 Issue 4, Nov. 1989 http://dl.acm.org.prox.lib.ncsu.edu/citation.cfm?id=75104.75105&coll=DL&dl=GUIDE&CFID=251927&CFTOKEN=71004880</ref>
In contrast, in a shared virtual memory system on a loosely coupled multiprocessor which has no physical shared memory (with a nontrivial communication cost between processors), conflicts are not likely to be solved with negligible delay. These conflicts resemble a “page fault” in a traditional virtual memory system. Thus, there are two design choices that greatly influence the implementation of a shared virtual memory: the granularity of the memory units (i.e., the “page size”) and the strategy for maintaining coherence.
Memory coherence strategies are classified based on how they deal with page synchronization and page ownership. The algorithms for memory coherence depend on the page fault handlers, their servers and the data structures used. So page table becomes an important part of these protocols.
Centralized Manager Algorithms
One way to obtain mutual exclusive access to data is to use a centralized algorithm. The first version of this algorithm is very similar to a monitor and makes use of a info table which has an entry for every page, and also three fields for each of those pages. The owner field keeps track of the processor which had the latest write access to the page. The copy set field has a list of every processor with a copy of the page, which is used for broadcast-free invalidation operations. Finally, the lock field is used when synchronizing requests for the page. Each processor in the algorithm also keeps track of page accessibility in its own page table with an access and a lock field.
By setting the lock in both of the tables, the algorithm can synchronize page faults whenever there are multiple processes waiting for a page, or whenever the page is in the process of being accessed. In conjunction with this are confirmation messages that are sent to let the managing processor know when it can give page access to someone else. So, while read-page faults on the managing processor only need a message to and a message from the owner, read-page faults on the non-managing processor need an additional message to the manager and a confirmation message. For write-page faults, the message sending process is the same, except for the additional cost of an invalidation.
The second version of the algorithm improves on the first by eliminating the need for confirmation messages. To accomplish this, the responsibility of synchronizing page ownership is moved from the manager to the individual owners. This also means that the lock field is removed from the info table, and the copy set field is transferred to each of the page tables. Ultimately, this removes the cost of one send and one receive from the algorithm.
Distributed Manager Algorithm
In order to fix the possibe bottle-neck centralized manager algorithms may create, Distributed Manager Algorithms assigns tasks to various individual processors. There are several methods of assigning these tasks. In a Fixed Distributed Manager Algorithm, there is a predetermined set of pages for which each processor is responsible. In a Broadcast Distributed Manager Algorithm, page faults results in broadcast requests to the other processors. Due to the number of requests, the Broadcast Distributed Manager Algorithm does not scale well. In the case of the Dynamic Distributed Manager Algorithm, instead of using broadcasting requests to find page owners, each processor holds a page table that stores the owners for each page. Since the processors that own each page (page owners) are not static, each entry in a processor's page table is only a "hint," or a starting point for looking for the true owner.
References
<References> [1] Yan Solihin. "Fundamentals of Parallel Computer Architecture: Multichip and Multicore Systems." Solihin Publishing & Consulting LLC, 2009.
[2] Sarita V. Adve. Kourosh Gharachorloo. "Shared Memory. Consistency Models: A Tutorial." Digital Western Research Laboratory 250 University Avenue Palo Alto. <http://www.hpl.hp.com/techreports/Compaq-DEC/WRL-95-7.pdf>
[3] Kai Li, and Paul Hudak. "Memory coherence in shared virtual memory systems". Published in Journal ACM Transactions on Computer Systems (TOCS), Volume 7 Issue 4, Nov. 1989 <http://dl.acm.org.prox.lib.ncsu.edu/citation.cfm?id=75104.75105&coll=DL&dl=GUIDE&CFID=251927&CFTOKEN=71004880>.
</References>
Quiz
1. Which of the following is true about Broadcast Distributed manager Algorithm? (More than one options could be correct)
a) It has a predetermined set of pages for which each processor is responsible.
b) Page faults results in broadcast requests to the other processors.
c) It does not scale well due to the number of requests.
d)None.
2.What is the major difference between One copy Serializabilty and Sequential consistency?
a) No difference
b) One copy Serializabilty Model has only one copy of the file.
c) Sequential Consistency Model has only one copy of the file.
d) None.
3.Which of the following is a hybrid protocol?
a) MSI
b) MOSI
c) Cachet
d) Firefly
4.On what basis are memory consistency strategies classified? (More than one options could be correct)
a) Page faults
b) Page synchronization
c) Page ownership
d) None.
5.Which of the following are the four states in a Dragon Protocol?
a) Modified, Shared, Invalidate
b) Modified, Exclusive, Shared Clean, and Shared Modified
c) Owned, Shared, Modified, Exclusive
d) None
6.Which of the following is true about sequential consistency? (More than one options could be correct)
a) All orderings are enforced.
b) Loads and stores can exchange positions freely.
c) Performance can suffer
d) SC is deterministic
7.In Centralized Manager Algorithms, what does the owner field signify?
a) Used when synchronizing requests for the page.
b) Keeps track of the processor which has the latest read access to the page.
c) Keeps track of the processor which has the latest write access to the page.
d) Entry for every page.
8.Which of the following is false? (More than one options could be correct)
a) Write Update method results in significant amount of traffic.
b) In a write through policy, the write is done only to the cache.
c) Cache coherence can be achieved by cache write policy.
d) Broadcast Distributed Manager Algorithm doesn’t scale well.
Quiz Answers: 1. b,c 2. b 3. c 4. b,c 5. b 6. a,c 7. c 8. a,d